electron-transport-chain-etc/
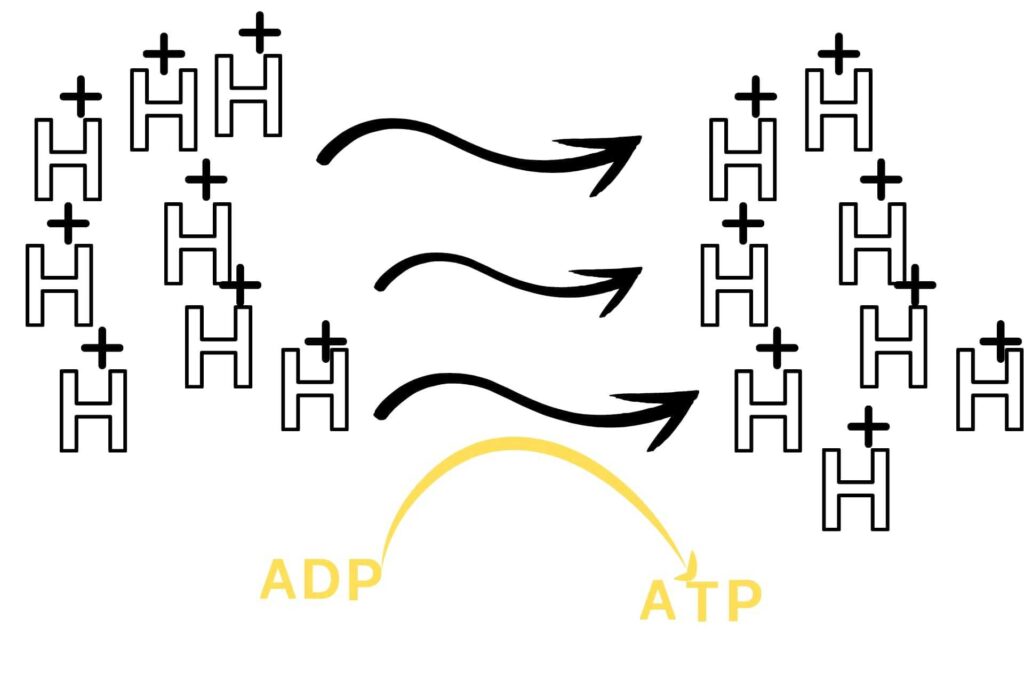
The electron transport chain (ETC) and oxidative phosphorylation are complex processes that are essential for the production of ATP, the body’s main energy currency. However, many people find Electron Transport Chain ETC and oxidative phosphorylation to be difficult to understand.
In this article, we will break down the ETC and oxidative phosphorylation into simple steps, each step with computer diagrams, and explain how they work. We will also discuss the relationship between the ETC and oxidative phosphorylation, glycolysis, and the Krebs cycle.
By the end of this article, you will have a clear understanding of ETC and oxidative phosphorylation and how they produce ATP. You will also be able to answer the question: “What is the relationship between the ETC and oxidative phosphorylation, glycolysis, and the Krebs cycle?”
First, we will start with Electron Transport Chain (ETC)
An electron transport chain is a chain of reactions in which an electron moves through a membrane system to release energy and that energy is used to convert ADP into ATP.
But what is oxidative phosphorylation?
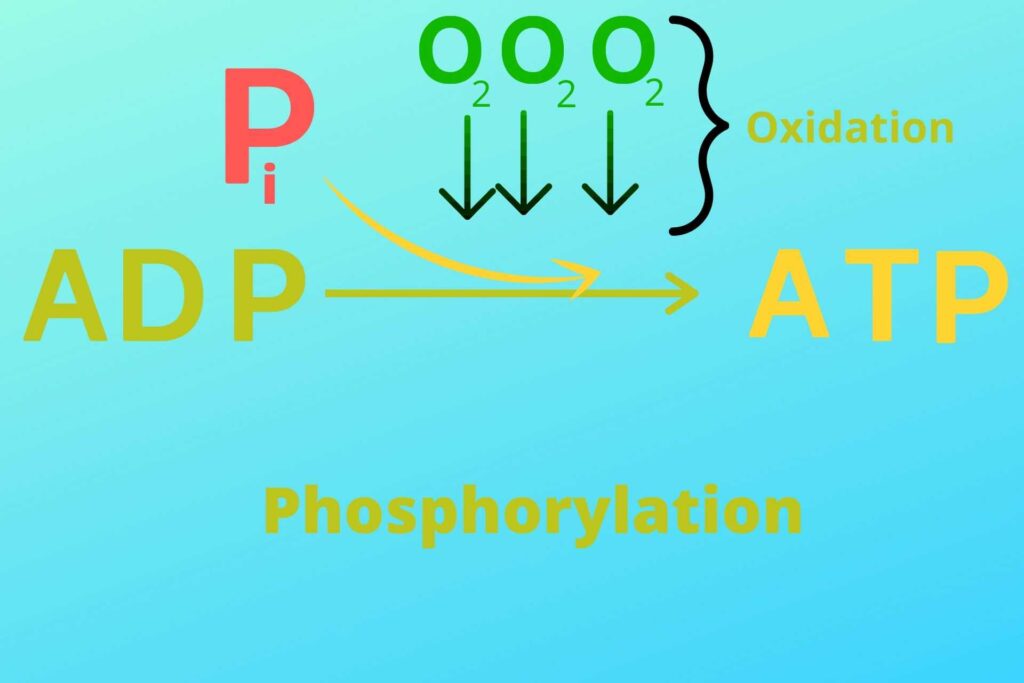
Phosphorylation means the addition of inorganic Phosphate to ADP while oxidative means in the presence of oxygen.
Oxygen? What is the role of oxygen in the electron transport chain?
As you know in the reaction of respiration, water (H2O) is produced. This water is produced because, at the end of the Electron transport chain, the Oxygen reacts with Hydrogen in mitochondria and makes water. It will be discussed in detail later in this article.
Why both are discussed in one article?
Because both have a link, which will be discussed later, just remember this question.
I would like to review back the previous process very shortly i.e. glycolysis and Krebs cycle.
In glycolysis, glucose (carbohydrates), Amino Acid (Protein), fatty acid, and glycerol (fats) are broken down to Acetyl Coenzyme A which moves in the Krebs cycle to produce energy-rich molecules (NADH and FADH2). These molecules then move to Electron Transport Chain and Oxidative Phosphorylation.
Have you thought that from where the Hydrogen comes to NADH and FADH2?
No.
Do you remember how “carbon” was removed from the molecule in the glycolysis and Krebs cycle?
Yes, that was through decarboxylation (removal of CO2).
That is correct.
In those processes along with CO2 hydrogen is also released which is loaded on NAD+ and FAD (coenzymes) and then these Coenzymes are converted into NADH+H2 and FADH2. The enzyme which is responsible for the removal of hydrogen is called dehydrogenase.
But NADH has extra hydrogen (NADH+H+), what is this then?
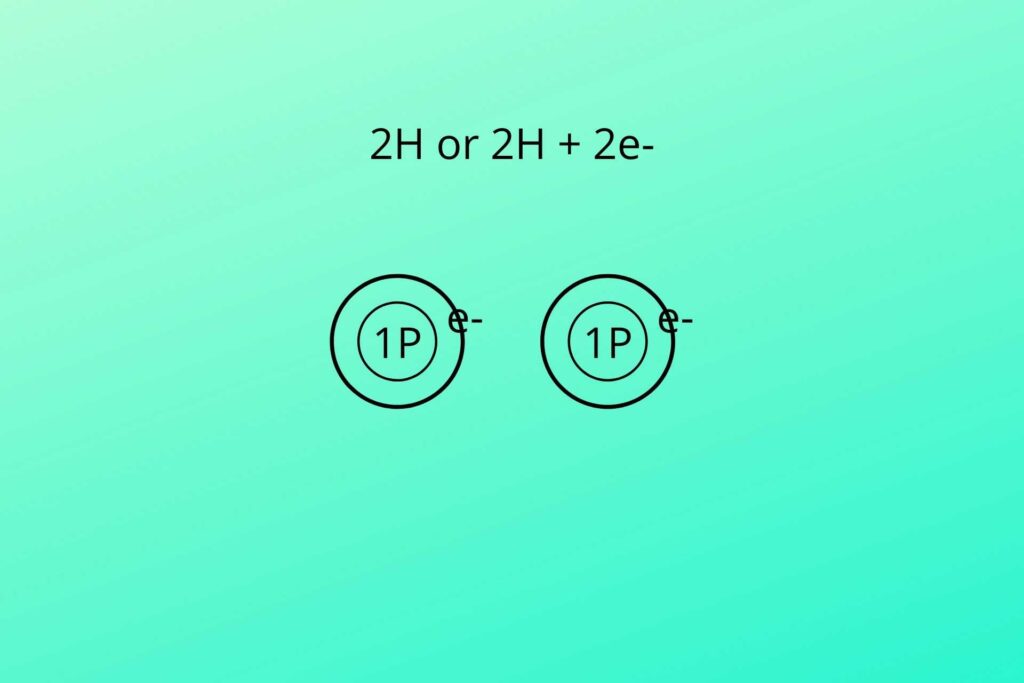
In glycolysis and Kreb’s cycle, the NAD+ and FAD+ are loaded with hydrogen. The hydrogen can be written as shown in the picture.
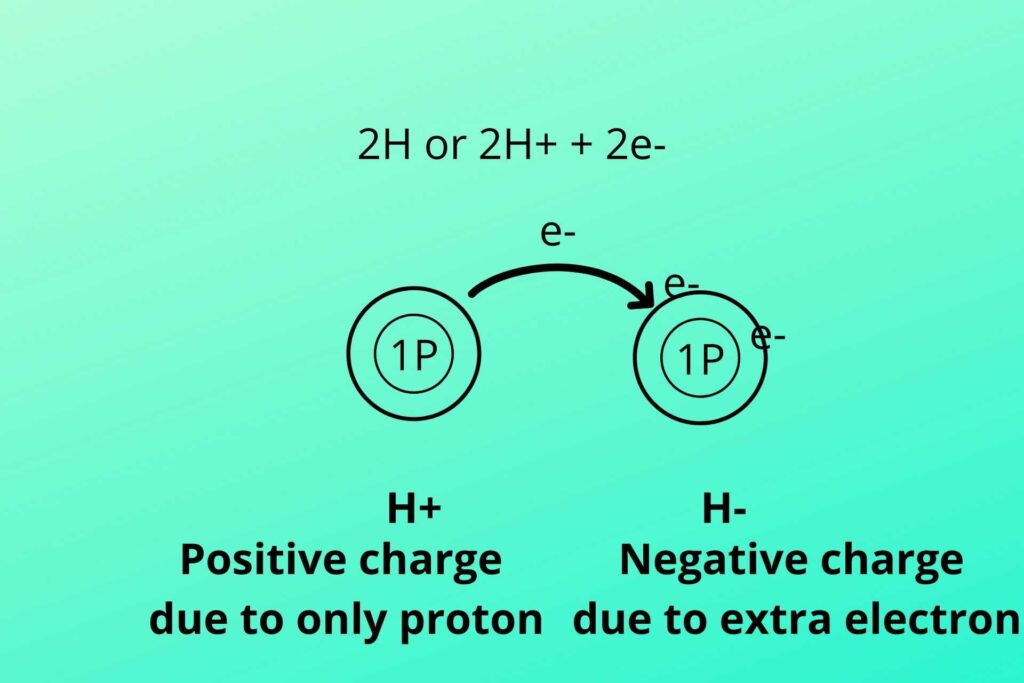
The electron from one hydrogen moves to another hydrogen which makes it negatively charge due to extra electron while the other becomes deprived of electron and become positively charged produced in glycolysis and Kreb’s cycle.
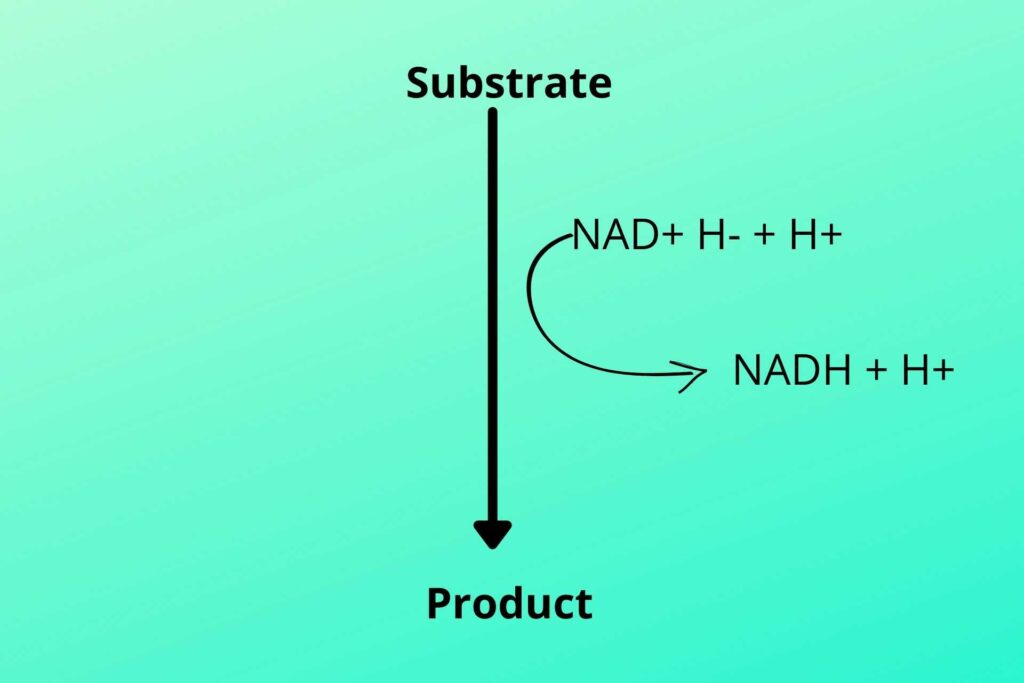
As previously discussed that two hydrogen atoms were produced, one with two electrons and one with no electron as shown in the picture. The negative charge hydrogen (due to extra electron) atom binds with NAD+ to convert it to NADH while the positive charge hydrogen (proton) atom is free (NADH+H+).
The same is the case for FAD but the only difference is that two hydrogens are added to convert it to FADH2.
The Electron Transport Chain (ETC) will extract electrons from NADH and FADH2.
Where is the electron?
As you know Hydrogen has one proton and one electron so when two Hydrogen are removed from the molecule the electron from the hydrogen atom shift to another hydrogen atom. In this way, one hydrogen atom has one extra electron i.e. two electrons while the other has no electron.
So it means the extra electron hydrogen atom is an electron?
Exactly, the extra electron hydrogen atom is called a hydride which is called an electron that will run in the Electron Transport Chain.
But can you tell us where this process (Electron Transport Chain) is located or takes place?
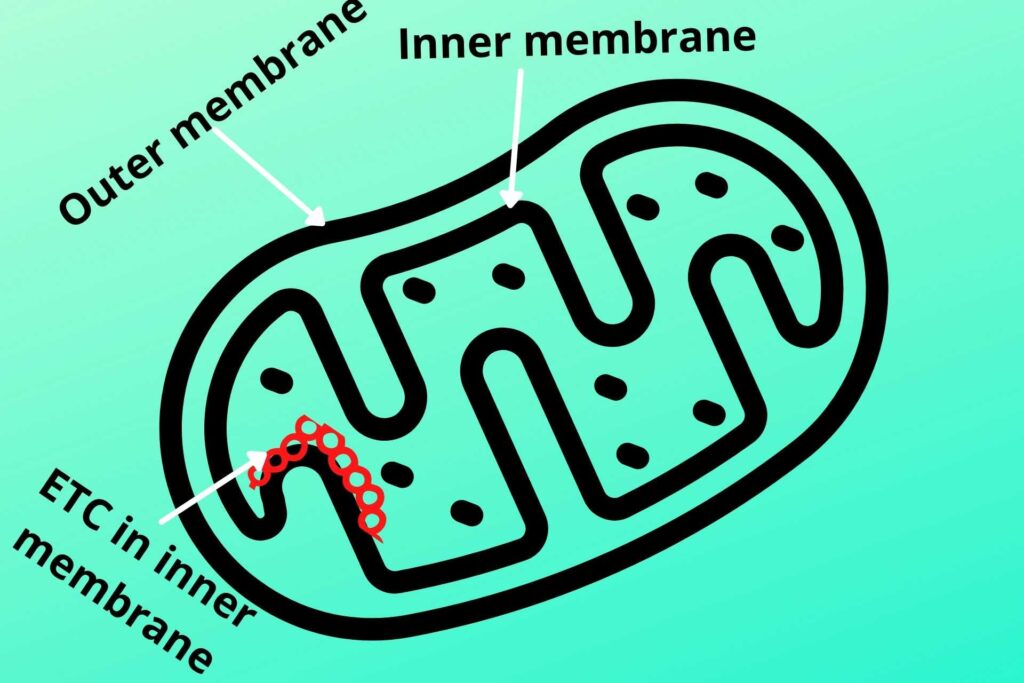
As already discussed glycolysis occurs in the cytosol while the Krebs cycle occurs in the mitochondrial matrix but Electron Transport Chain (ETC) occurs in the inner mitochondrial membrane.
Let me tell you briefly about the structure of mitochondria.
The inner side of the inner membrane of mitochondria has special protein complexes except for Coenzyme Q (not a protein) called Electron Transport Chain (ETC) where electron moves and energy are released.
There are another important structure and protein complex called the inner mitochondrial particle which is responsible for the synthesis of ATP. The inner membrane has a lot of protein carriers for the transport of different molecules but the inner membrane is relatively less permeable than the outer mitochondrial membrane.
From where do the steps of a proper Electron Transport Chain (ETC) start?
Let’s start with the proper steps of ETC.
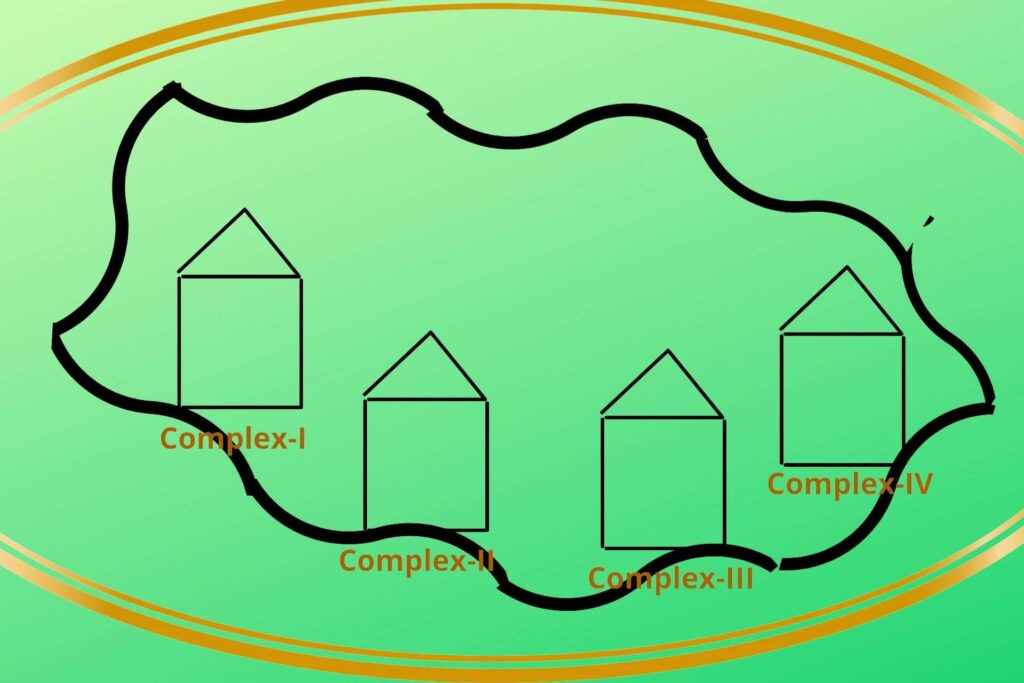
There are four protein complexes in Electron Transport Chain or system (ETC) namely Complex-I, Complex-II, Complex-III, and Complex-IV respectively. These protein complexes are fixed on their place in the inner membrane of mitochondria.
Step 1:
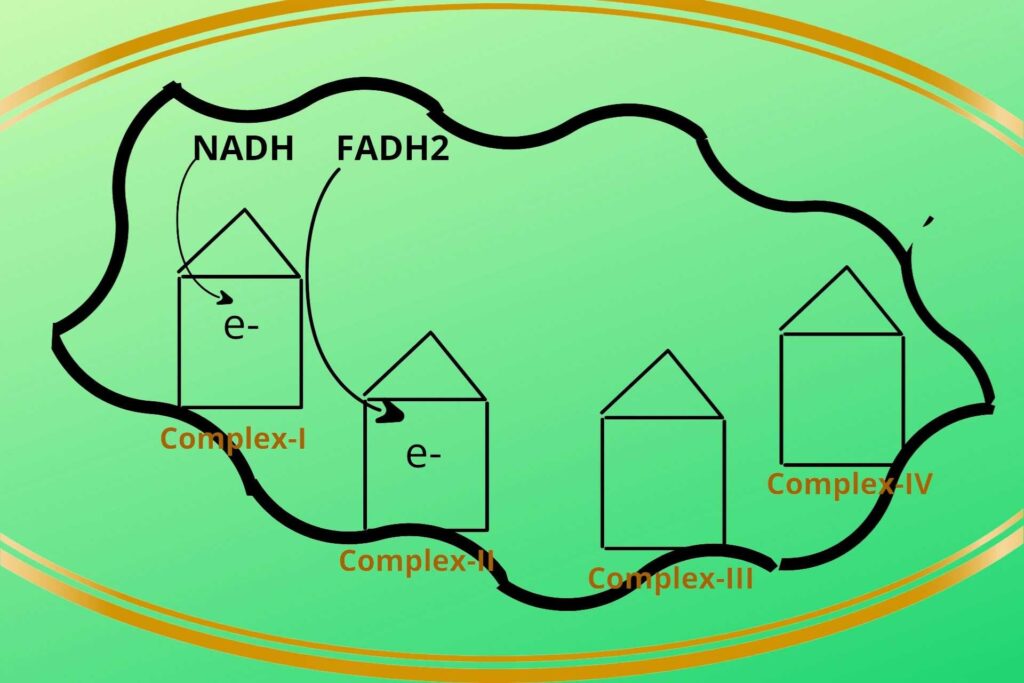
Then the NADH+H+ loads/give their electrons (electron means Hydrogen with extra electron i.e H-) to complex-I and gets oxidized back to NAD+. Similarly, the FADH2 gives its electrons to Complex-II and gets oxidized back to FAD.
From where does the NADH come?
As explained NADH is produced in glycolysis and Krebs cycle. The NADH of the Krebs cycle easily enters to Electron Transport Chain (ETC) but there is a problem with NADH of glycolysis.
What is the problem?
The problem is that the NADH of glycolysis (present in the cytosol) can’t enter mitochondria from cytosol because the inner membrane of mitochondria is impermeable to it.
So now how will the NADH enter?
The NADH will give their Hydrogen to oxaloacetate in the cytoplasm which is converted to Malate. The Malate now can enter mitochondria.
After entry what will happen to it?
The Malate is again converted to Oxaloacetate in mitochondria and the energy released during their conversion is utilized to convert mitochondrial NAD+ into NADH and this NADH is then given to Complex-I. By this method, the cytosolic NADH enters mitochondria for Electron Transport Chain (ETC).
But here there is again one problem.
What?
Oxaloacetate can’t directly come out from mitochondria so now they are trapped here.
But what they will do to come out?
The Oxaloacetate combines with glutamate and is then broken down into α-keto glutaric acid and Aspartate, now these two molecules are allowed to leave mitochondria. The α-keto glutaric acid and Aspartate again combine together in the cytosol to form glutamate which can enter the mitochondria.
But how the electrons are taken by complex-I?
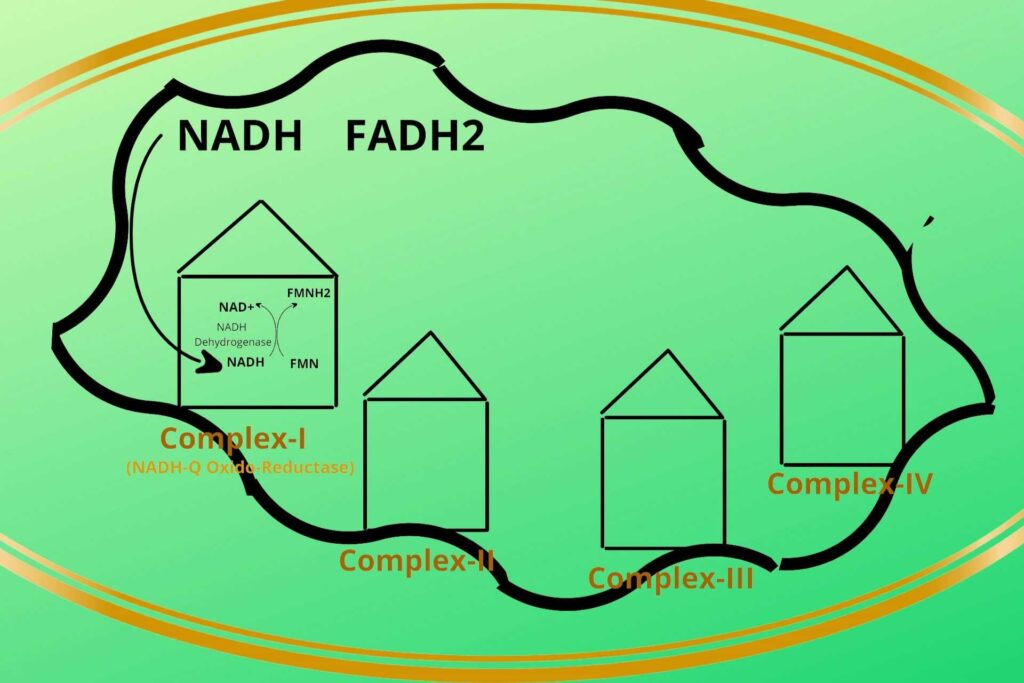
In complex-I, there is an enzyme called NADH dehydrogenase which takes the electron from NADH and gives it to another molecule called FMN which after taking the electron becomes FMNH2. Complex-I has been given a special name called NADH-Q Oxido-Reductase.
Why the name is so difficult?
Don’t worry it’s a logical name. In complex-I NADH is oxidized to release electrons while Coenzyme Q is reduced because it gains electrons, that’s why it is given the name NADH-Q Oxido-Reductase.
Let me shortly define, oxidation and reduction so that your concept is clear.
Oxidation:
Oxidation is the reaction in which any one of the following processes occurs:
4 Fe + 3O2 2Fe2O3
i) Addition of oxygen (O2) to the molecule in a reaction.
2NH3 + 3Br2 N2 + 6HBr
ii) Loss of Hydrogen (H2).
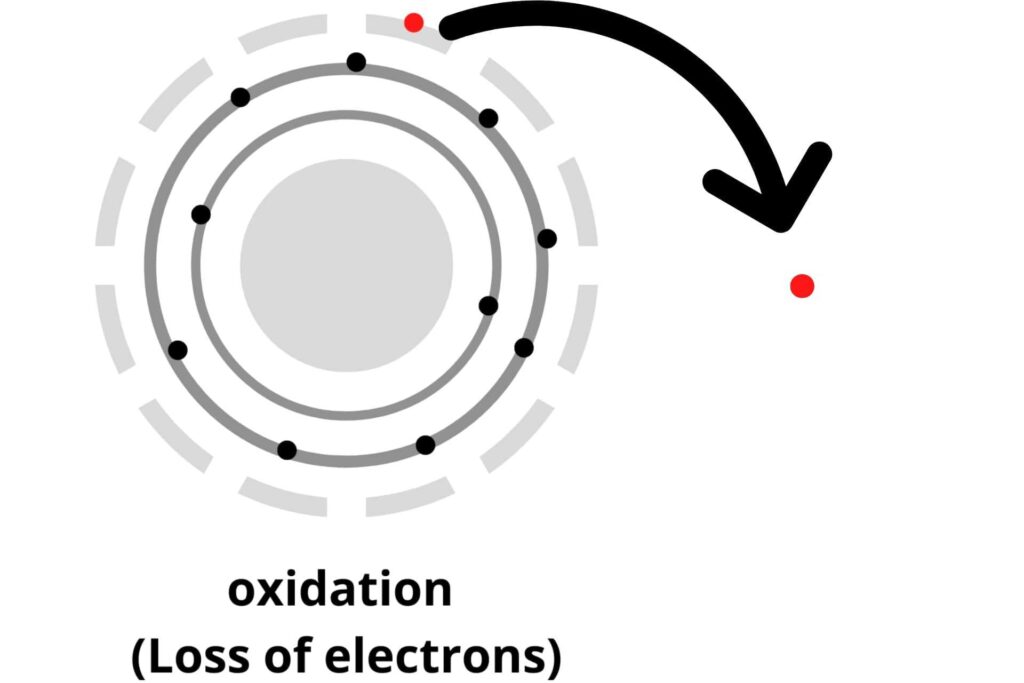
iii) And the loss of electrons.
Reduction:
The reduction is opposite to oxidation i.e:
Fe2O3 + 3CO 2Fe + 3CO2
i) Loss of oxygen. (But here we can also see that oxygen is taken by carbon monoxide (3co) and become carbon dioxide (Co2) which is an oxidation reaction. This type of reaction where both oxidation and reduction occur is called the Redox reaction or Oxidation-Reduction reaction.)
Oxidation (loss of Hydrogen)
2NH3 + 3Br2 N2 + 6HBr
ii) Addition of Hydrogen.
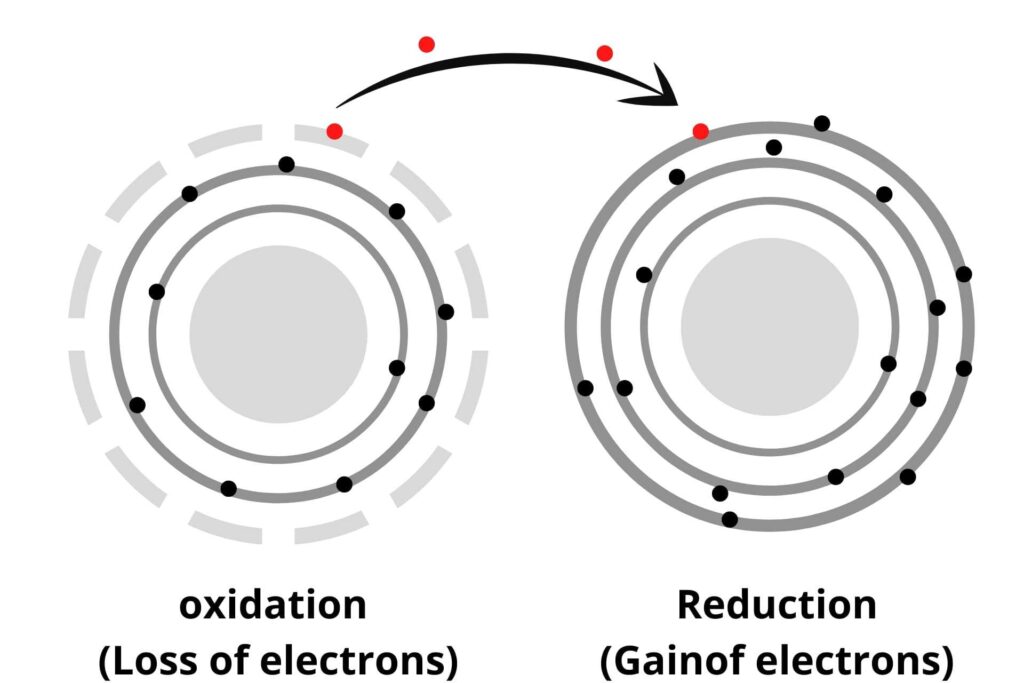
iii) Addition of electrons.
The electron is then transferred to the next complex.
But you told me previously that these complexes are fixed to their position then how these electrons are transported to the next complexes?
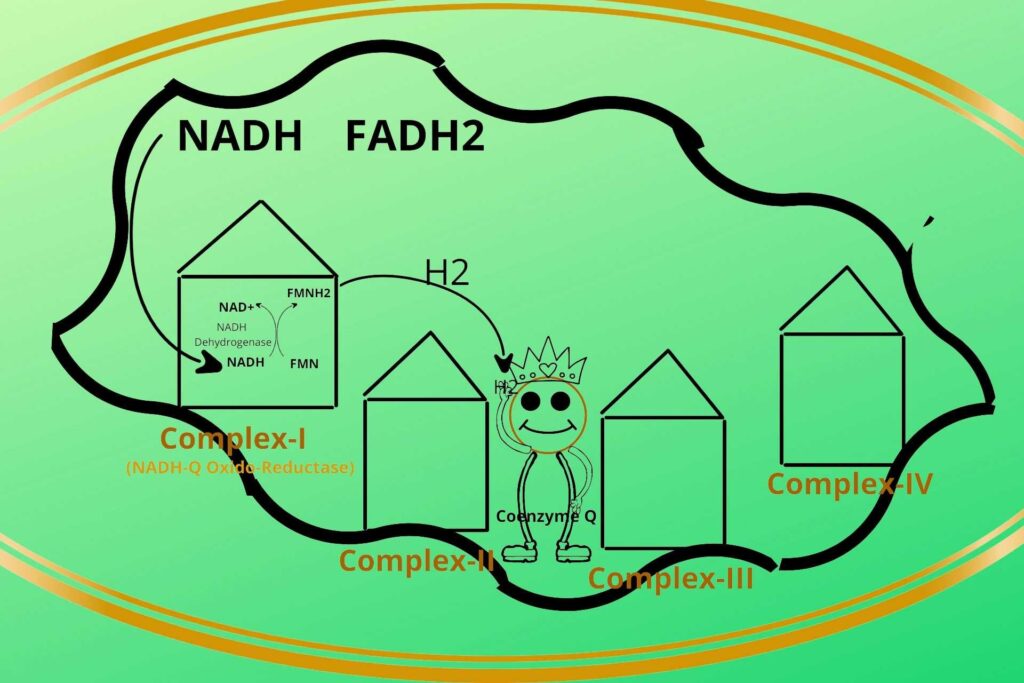
Well, the electrons from FMNH2 in complex-I (NADH-Q Oxido-Reductase) are given to a non-protein carrier called Coenzyme Q and are transported to Complex-III.
Why it is not transported to Complex II?
Because Complex-II receives electrons from FADH2, that’s why complex-I electrons are directly transported to Complex-III through Coenzyme Q.
But what does the Complex-I do with that energy?
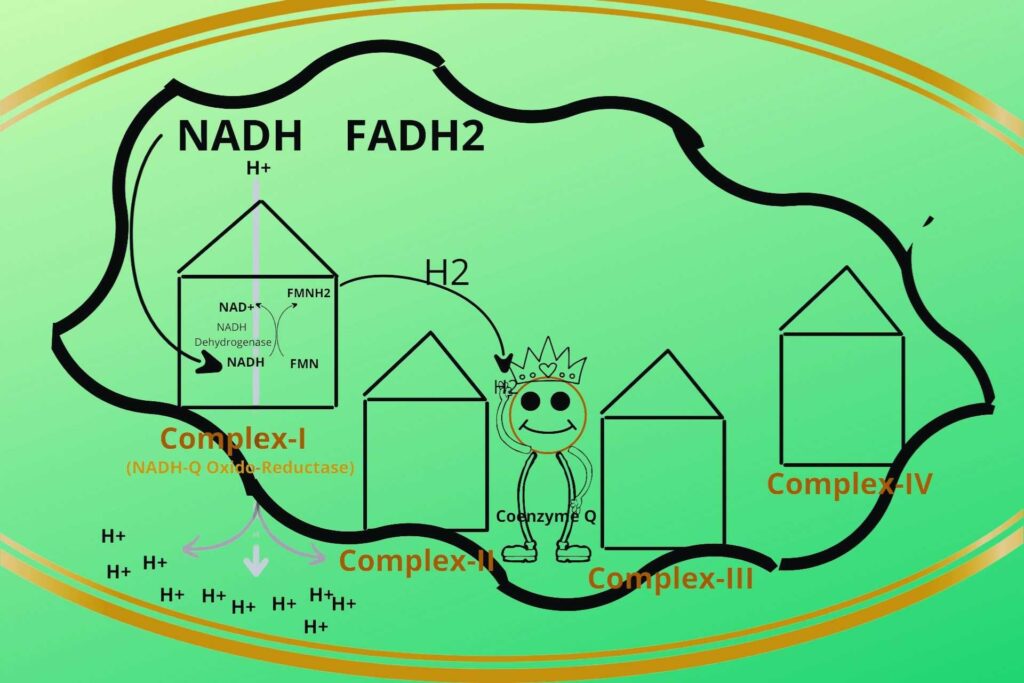
The complex-I then uses that energy to transfer the H+ ions from the mitochondrial matrix to the intermembranous space of mitochondria.
Step 2:
Step II starts from Complex II. Before going to this let me ask you a question.
Do you remember step-6 of the Krebs cycle?
I have to go back to it to remind it.
Let me tell you.
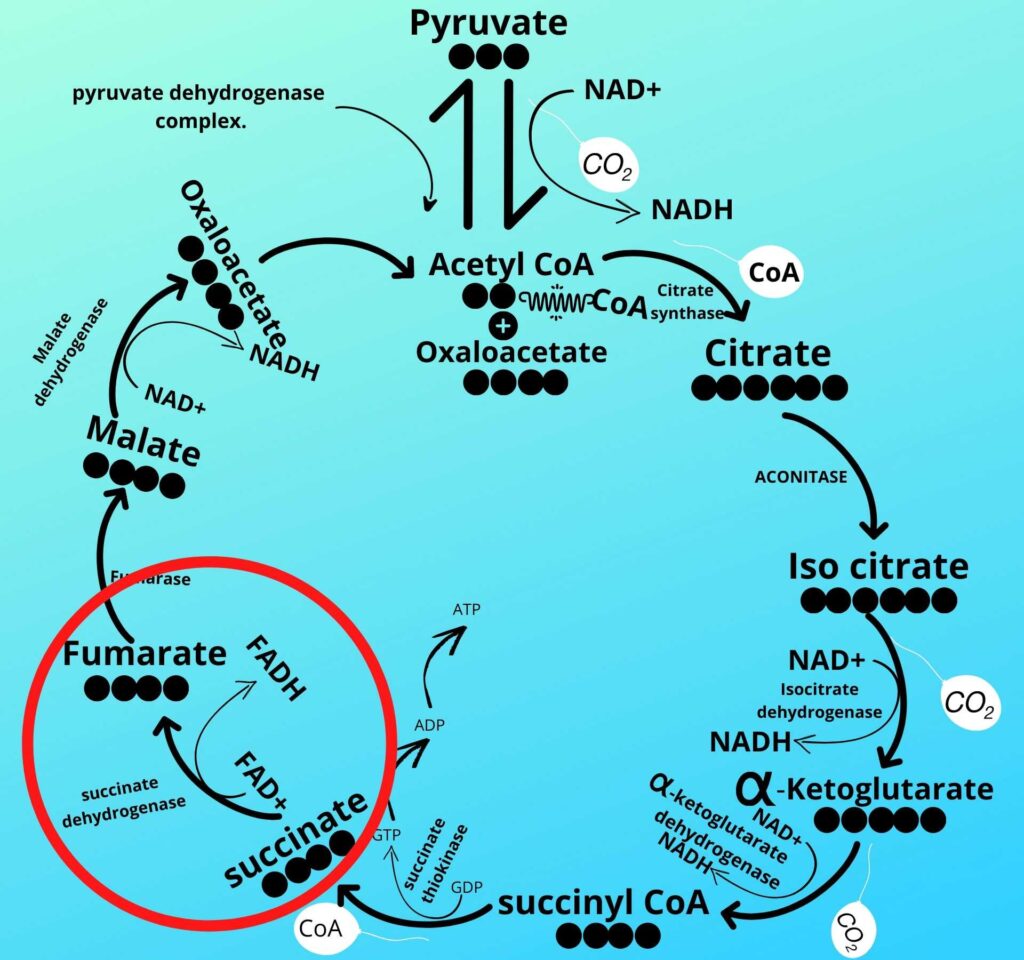
As already discussed in step-6 of the Krebs cycle succinate dehydrogenase converts succinate into Fumarate by removing Hydrogen from it and adding that Hydrogen to coenzyme FAD releasing FADH2.
What is the relation of this step here?
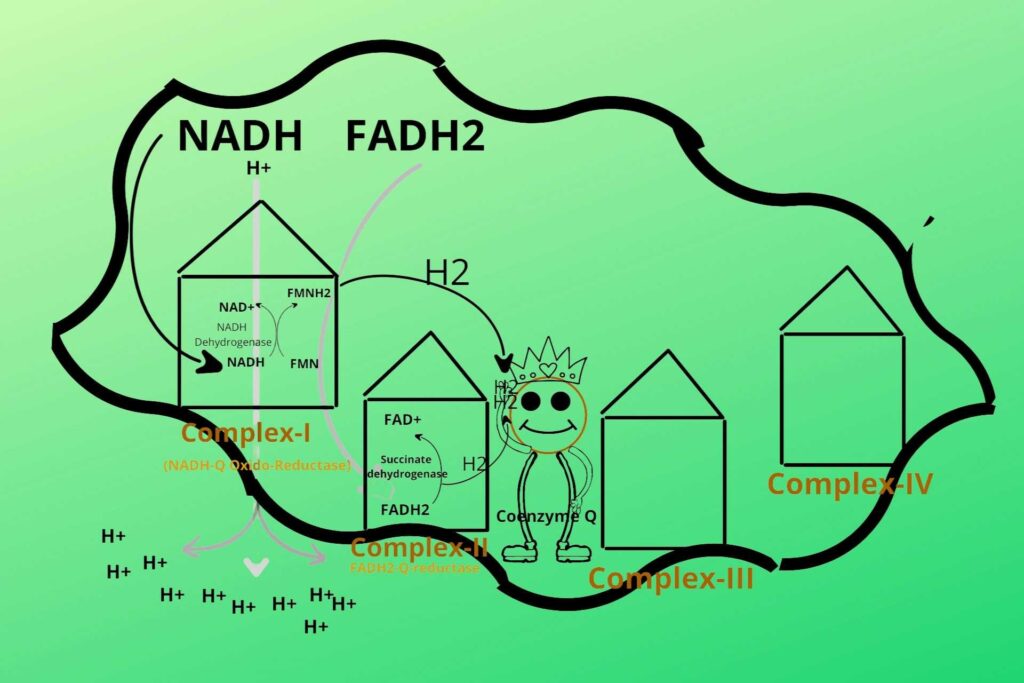
Because the succinate dehydrogenase enzyme is also present here (Complex-II). succinate dehydrogenase removes Hydrogen (electron) from FADH2 and gives it to Coenzyme Q. FADH2 is converted back to FAD after the removal of an electron from it and ready for the process again. Complex II is called FADH2-Q-reductase. But remember that complex-II doesn’t release hydrogen ions from the mitochondrial matrix to inter membranous space because it directly gives their electrons to Coenzyme Q. After all, their energy is less to do this.
So what will do Coenzyme Q after this?
Wait, a little because Coenzyme Q receives more electrons from the cytosol.
But how, because the inner membrane of mitochondria is impermeable to outside molecules?
Nature has created every process perfectly. Let me ask you again a question here before going into details.
Okay.
Do you remember step-4 of glycolysis?
Can you repeat here, Please?
Okay, don’t worry.
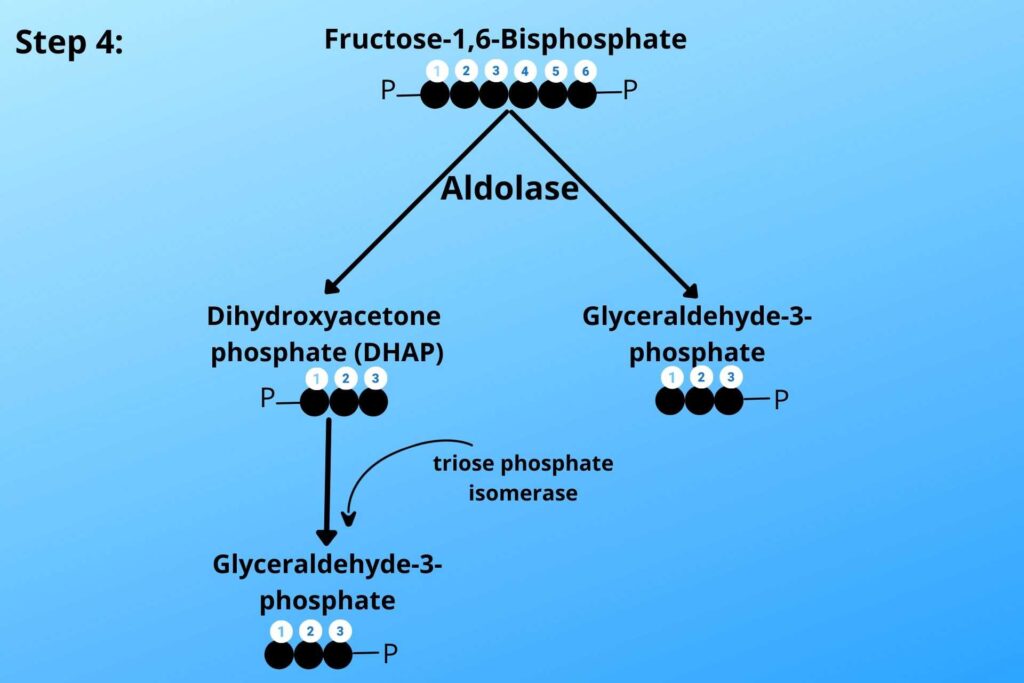
In step-4 of glycolysis dihydroxyacetone phosphate (DHAP) is converted to Glyceraldehyde-3-phosphate.
Yes.
And you also know that glycolysis also produces some NADH in the cytosol as discussed earlier?
Yes, I know that.
And you also know that NADH cannot directly enter the inner membrane of mitochondria!
Yes, I know this too.
But do you know how this NADH will enter mitochondria?
Am, that’s the point.
Can you tell us how this NADH will enter mitochondria?
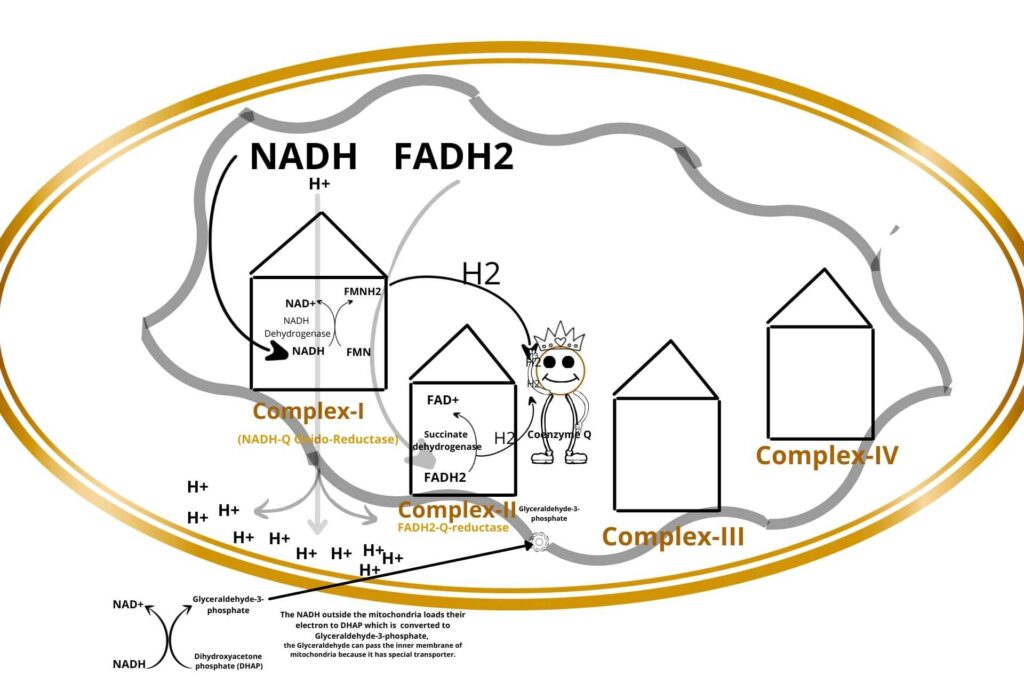
During the conversion of dihydroxyacetone phosphate (DHAP) to Glyceraldehyde-3-phosphate, some of the NADH binds with Glyceraldehyde-3-phosphate, now the Glyceraldehyde-3-phosphate can enter the inner membrane of mitochondria to Electron Transport Chain (ETC) because Glyceraldehyde-3-phosphate has a special transport carrier on the inner membrane of mitochondria.
Now, what happened to Glyceraldehyde-3-phosphate in mitochondria?
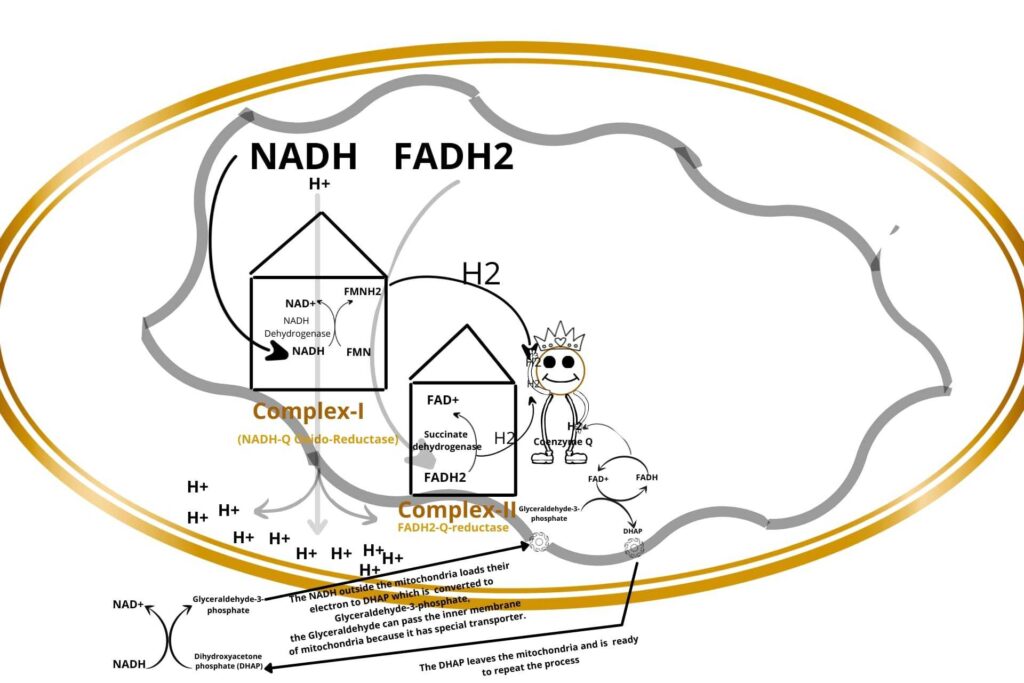
The Glyceraldehyde-3-phosphate is again converted back to dihydroxyacetone phosphate (DHAP) but the energy released here is used to convert FAD to FADH2. The FADH2 release their electron to Coenzyme Q and are reduced. The dihydroxyacetone phosphate (DHAP) now leaves the mitochondria.
So it means the reduced Coenzyme Q can receive electrons from multiple points i.e. Complex-I, Complex-II, and Cytosol.
Step-3:
In step-3 the electrons are given to complex-III. In complex III, there is a protein called cytochrome-b.
Do you know cytochrome?
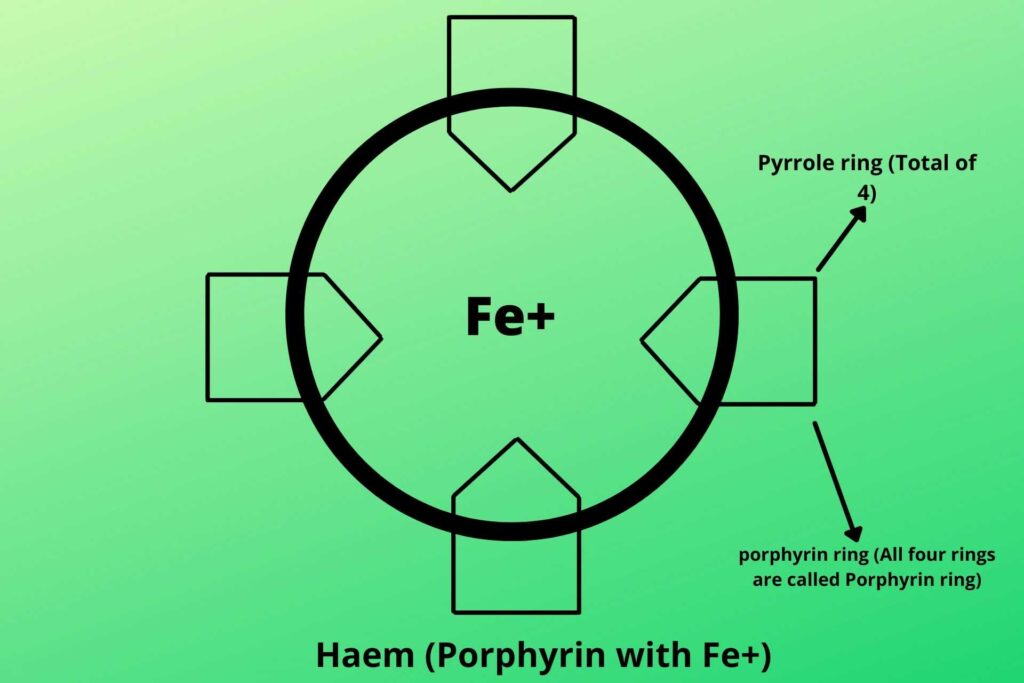
Cytochrome is a special protein that has the iron group in the middle with the surrounding porphyrin ring. A porphyrin ring is the combination of four pyrrole rings that makes a ring and with iron in the middle, it is called haem.
Is this haem group the same group that is present in hemoglobin?
Yes, but besides hemoglobin, it is also present in cytochromes, and enzymes called catalases.
The electrons are going to be accepted by cytochrome-b in complex-III?
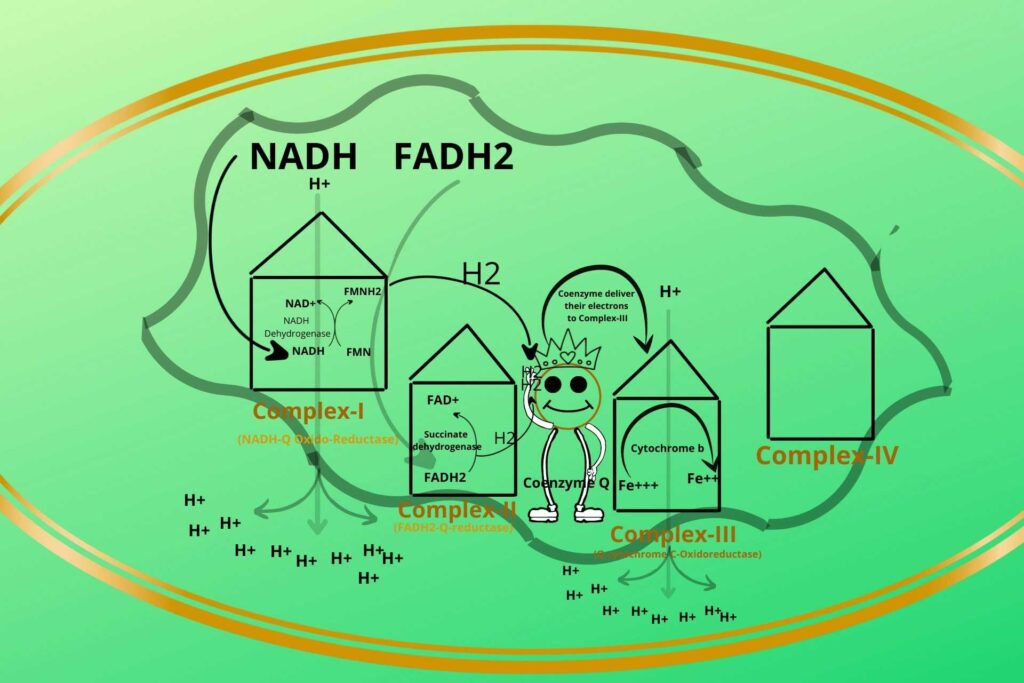
Yes, but before going to this you should know that cytochrome-b has iron in ferric (Fe+++) and ferrous (Fe++) forms. The Coenzyme Q now comes to complex-III and delivers its electron to Ferric iron (Fe+++) (oxidized) which becomes Ferrous iron ion (Fe++) (reduced).
Can the Ferrous ion go back to its Ferric form?
Yes, by losing the accepted electron.
How?
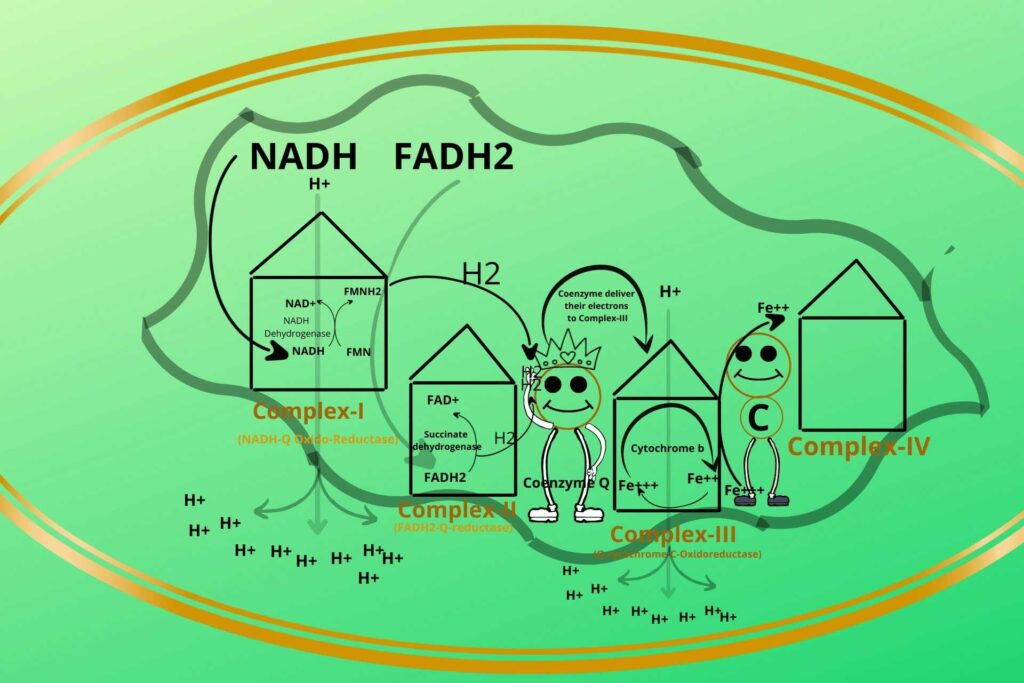
There is cytochrome-c present between complex-III and complex-IV which takes the electron from a Ferrous ion and converts it back to a Ferric ion while cytochrome-c has itself Ferric (Fe+++) and Ferrous ions, the Ferric ion (Fe+++) gets reduced to Ferrous (Fe++) after taking electrons from Complex-III.
There must be the release of energy during this process?
Of course and that energy is used to transfer Hydrogen ions from the mitochondrial matrix to the inter membranous space of mitochondria.
Based on the process that occurred in complex-III it has been given a special name called Q-cytochrome-C-Oxidoreductase.
Why this name is given?
Because cytochrome Q is transferring its electrons to Cytochrome C that’s why the name Q-cytochrome-C is given while the oxidoreductase means there is an oxidation-reduction reaction between these.
Okay.
Step-4:
Step-4 starts with complex IV. In this step, the transfer of electrons from Cytochrome-C to complex-Iv occurs.
How?
Complex-IV has another type of cytochrome called Cytochrome A+A3.
What is Cytochrome A+A3?
The only difference between cytochrome A+A3 from Cytochrome b and c is that Cytochrome A+A3 has iron (Fe++) as well as copper (cu++)ions.
Now, what happened to electrons in this complex?
Do you remember that water (H2O) is produced during respiration?
C6H12O6 + 6H2O 6CO2 + 12H2O + ENERGY
Yes, I see in the reaction.
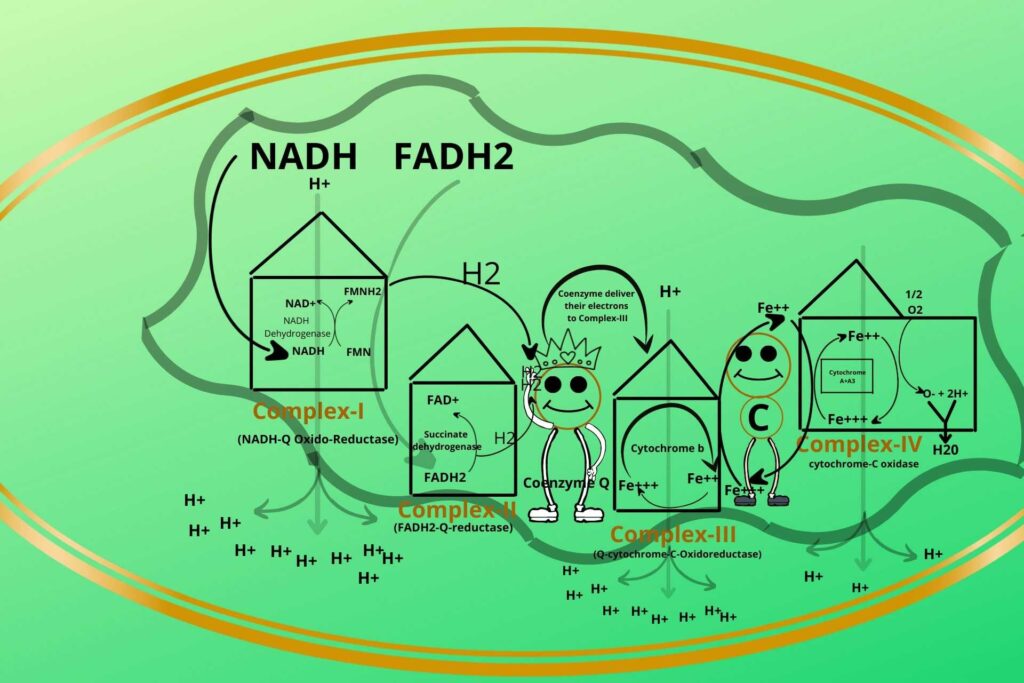
These electrons are given by Complex-IV i.e. Cytochrome A+A3 to molecular stable oxygen (O2) to make it negatively unstable charged oxygen (O–).
So now oxygen will react?
Exactly, the negatively charged oxygen is now highly reactive so it will react with Hydrogen present in mitochondria to make water (H2O).
The special name given to complex-IV is cytochrome-C oxidase.
So what did you learn?
Let me shortly repeat you. When electrons are moving from complex-I to Complex-IV it releases their energy to these complexes. The complexes then use this energy and transfer the hydrogen ions from the mitochondrial matrix to inter membranous space and electrons get very low in energy during this movement which in the end react with oxygen and make water.
But I didn’t see where ATP is produced?
Excellent question.
You have learned that during the electron transport chain, hydrogen ions are transported from the matrix to intermembranous space?
Yes.
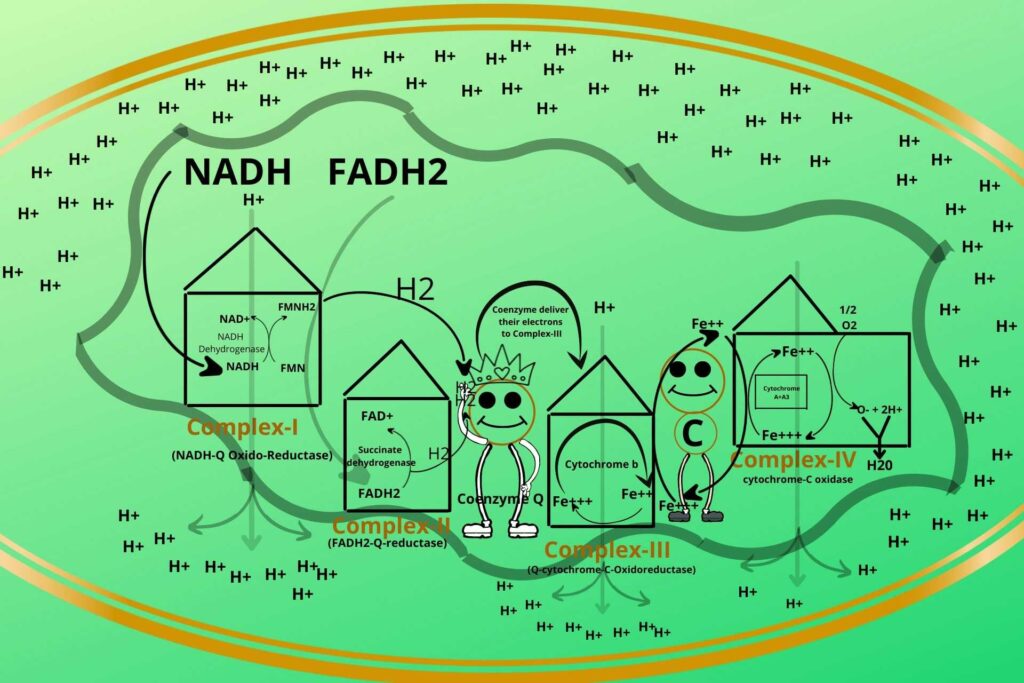
So after transportation, the concentration of Hydrogen ions increases in inter membranous space.
What do you think, what gonna happen?
Can you tell us?
The high concentration of hydrogen ions creates a potential gradient so the molecules will diffuse from higher concentration (inter membranous space) to lower concentration (inner matrix) and also outside the hydrogen ions create electronegativity while the inner becomes electropositive so there is a force of attraction between them.
But how they will enter?
As you know inner membrane is not permeable to Hydrogen ions so there is another complex system called ATP synthetase through which hydrogen ions enter.
Let me tell you what is ATP synthetase?
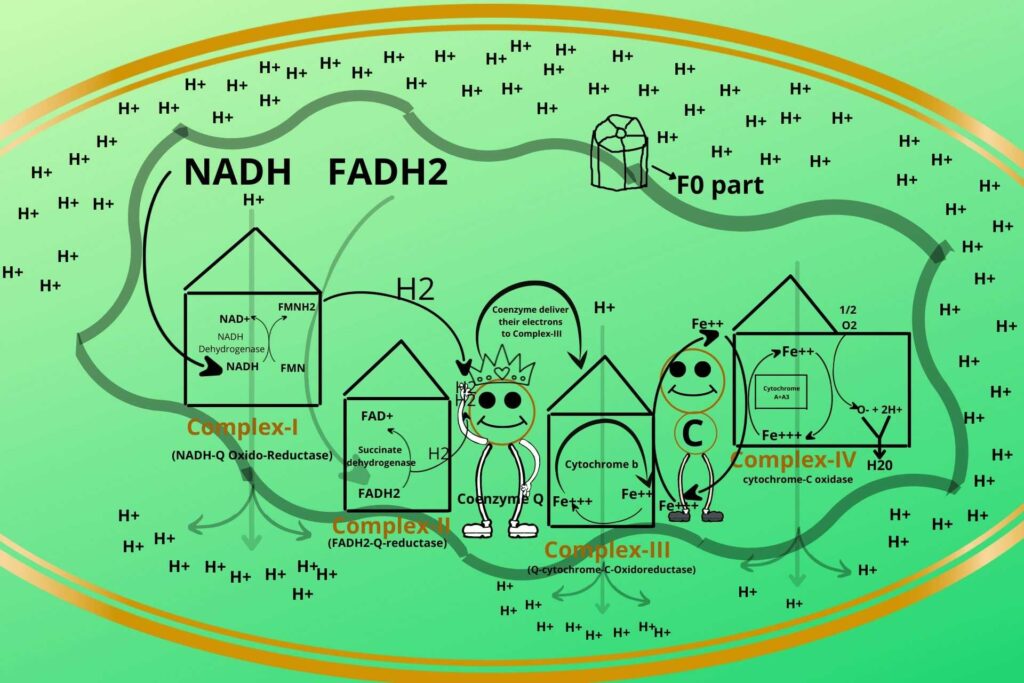
ATP Synthetase is a rotatory ring-like structure made of peptide rings arranged together to form a ring-like structure with a hole in the middle through which Hydrogen ions (protons) enter. This part of ATP Synthetase is called the F0 part.
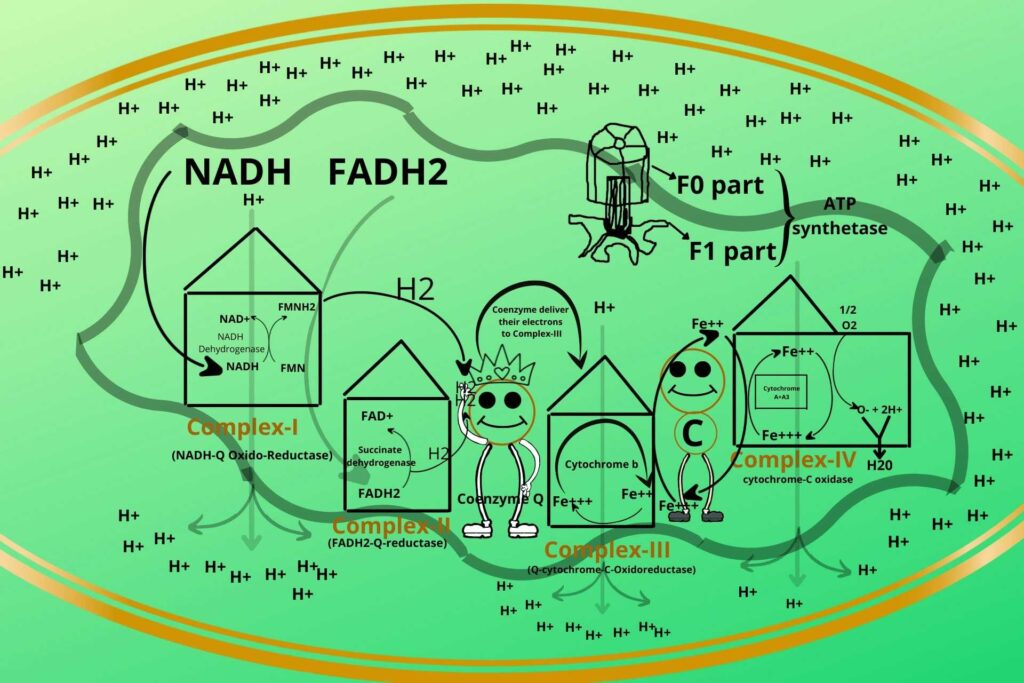
ATP Synthetase has another protein in its base called the F1 part which has a hook-like structure to its end as shown in the picture to which ADP and phosphate bind.
How does the process occur?
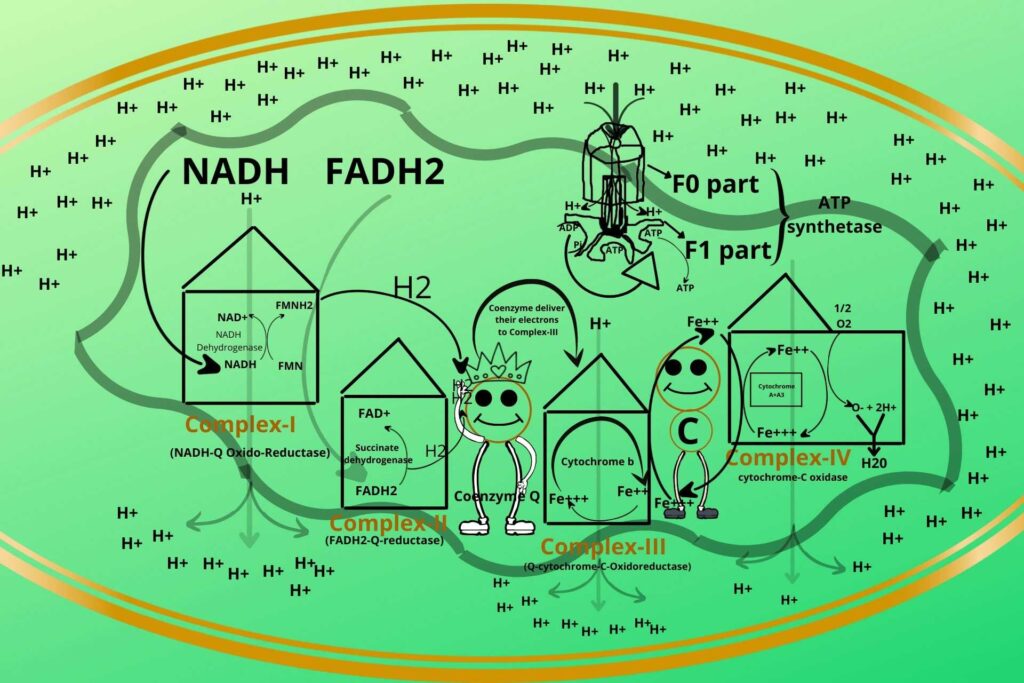
During entry of Hydrogen to the matrix through ATP synthetase, the ATP synthetase rotates, meanwhile, the ADP and phosphate bind to their binding sites at the base of ATP synthetase, when it rotates a little more the inorganic phosphate and ADP are closed in that binding sites and when it rotates even a little more the inorganic phosphate is added to ADP to make ATP which is released to the mitochondrial matrix.
This process continues and ATP is synthesized.
But here I want to tell you about one clinical situation?
Sure, please.
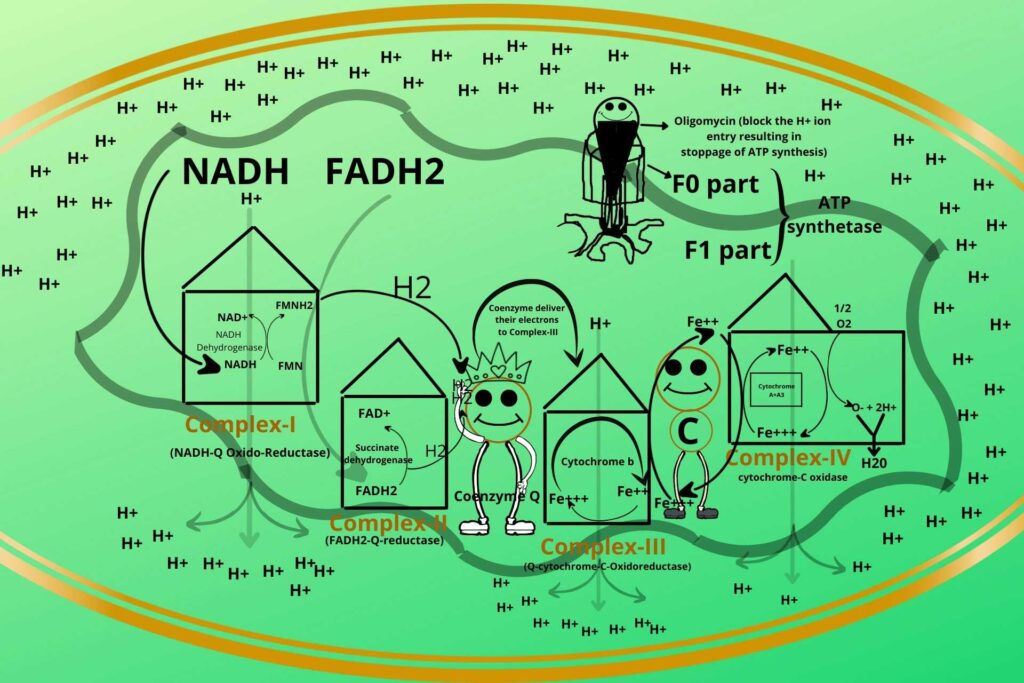
Sometimes in ATP synthetase, an inhibitor called Oligomycin comes and blocks the pathway due to which the entry of Hydrogen ions stops which results in the stoppage of ATP synthesis so great care should be taken during oligomycin taking.
But wait how does ADP enter mitochondria because the inner membrane is also impermeable to ADP?
You are a genius.
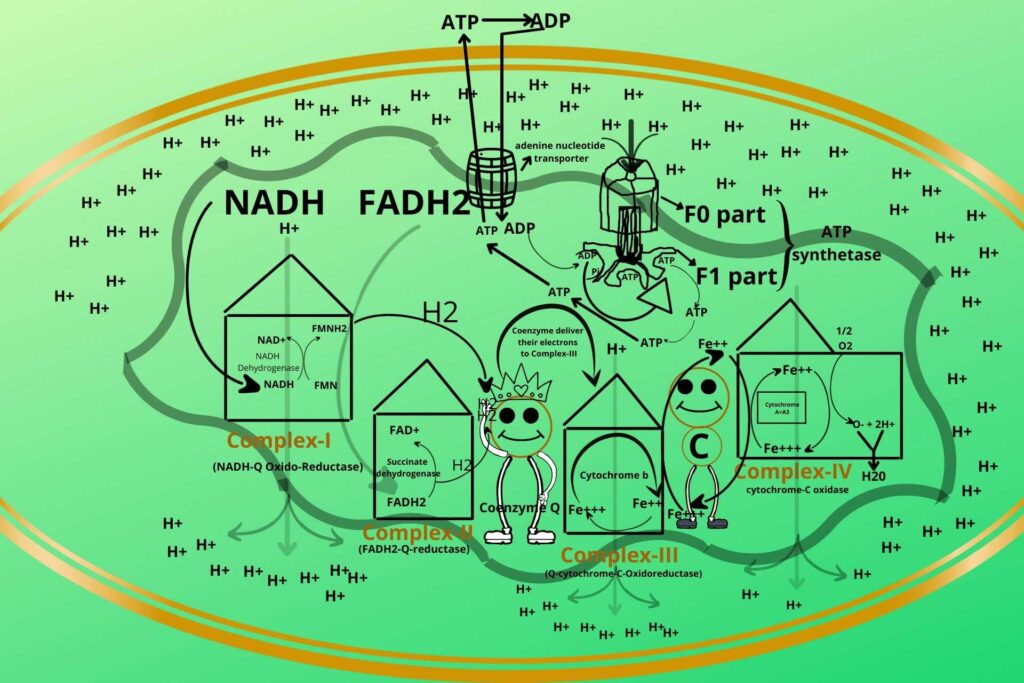
There is a special transporter for the transport of ADP from the cytoplasm to mitochondria and ATP to the cytoplasm called an adenine nucleotide transporter. The ADP enters through Adenine Nucleotide Transporter to mitochondria where phosphate is added at the F1 part of ATP Synthetase. After the formation of ATP, the ATP leaves the mitochondria through the same Adenine Nucleotide Transporter and is used to release energy in the cytosol and is converted into ADP which again enters mitochondria through the same transporter.
Here also I want to mention a medical situation about this transporter.
Atractyloside is a natural toxic inhibitor of Adenine Nucleotide Transporter due to which the entry of ADP block and when ADP block then surely ATP formation also stops so inhibiting the electron transport chain.
The whole process of movement of Hydrogen ions to intermembranous space and then diffusion of Hydrogen ions to the matrix through ATP Synthetase is called Chemo Osmotic Hypothesis of Oxidation-Reduction.
Now if I ask what is the relation between the electron transport chain and oxidative phosphorylation?
Still confused.
Do you know where oxidative phosphorylation takes place?
Yes, in mitochondria.
That’s correct.
And I am sure you know what happened during the electron transport chain?
Yes, there is the transport of electrons from different complexes with the release of energy.
That’s correct.
But what this process is called?
I think oxidation-reduction.
Exactly.
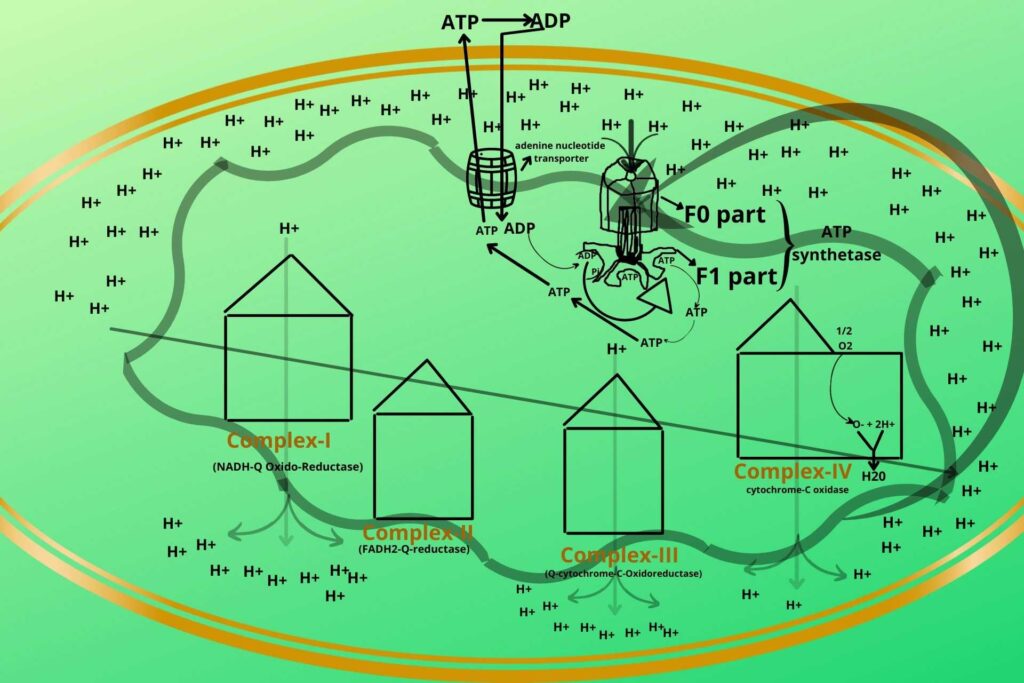
When NADH and FADH2 reach complex-I it delivers their electrons to it. It means the NADH and FADH2 oxidized while the complex-I is reduced. This chain reaction goes in the same way in which the first one loses its electron and gets oxidized while the receiver gets reduced until the H2O forms.
After that what happened?
After this, the Hydrogen ions get an increase in intermembranous space creating higher potential gradients which through diffusion the Hydrogen ions enter the matrix through ATP SYNTHETASE, and ATP is synthesized.
Did you get any idea?
A little.
Let me tell you.
The phosphorylation occurs due to oxidation in Electron Transport Chain (ETC) that’s why it is called Electron Transport Chain (ETC) and Oxidative Phosphorylation which also means Electron Transport Chain (ETC) and Oxidative Phosphorylation are coupled processes.
That’s clear now.
But here is another most important process that occurs, you should learn it!
And What it is?
There are some substances (Uncouplers) that uncouple both processes.
What is meant by uncoupling?
Previously you have learned the Electron Transport Chain (ETC) and Oxidative Phosphorylation both are coupled processes.
Yes.
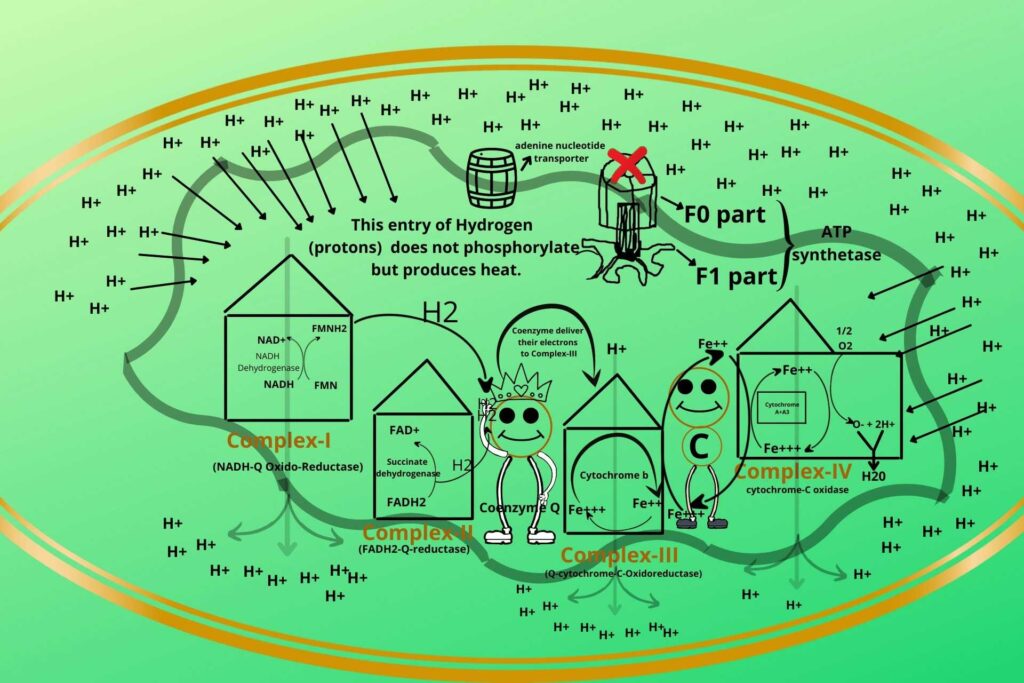
Uncouplers do the direct entry of Hydrogen ions without entering through ATP synthetase so in this case, the Electron Transport Chain (ETC) keeps working rather it becomes more active while Phosphorylation stops.
Will there be the production of energy?
Yes.
Where that energy goes?
This energy is used to produce heat and not ATP.
Do you know which substance is uncoupler in our daily life use medicines?
No.
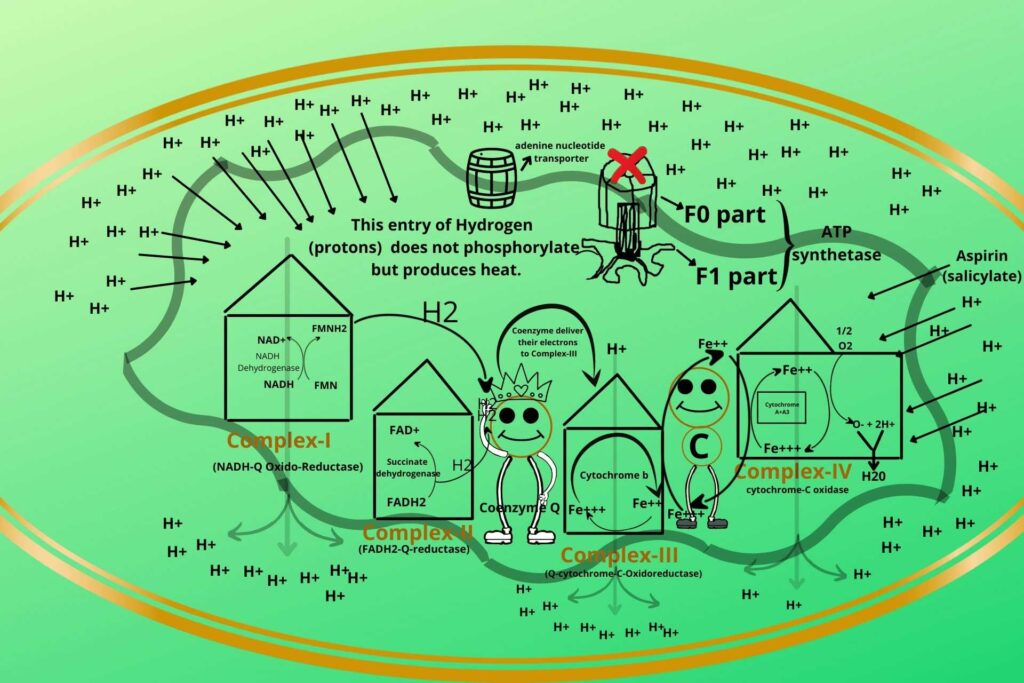
Aspirin (salicylate) is usually a fever reliever but a high concentration uncouples the process and produces heat so you should take great care while taking it.
In such a condition, the patient uses more oxygen due to which Electron Transport Chain (ETC) is stimulated more but there is less energy production.
But this uncoupling process has one benefit too.
How?
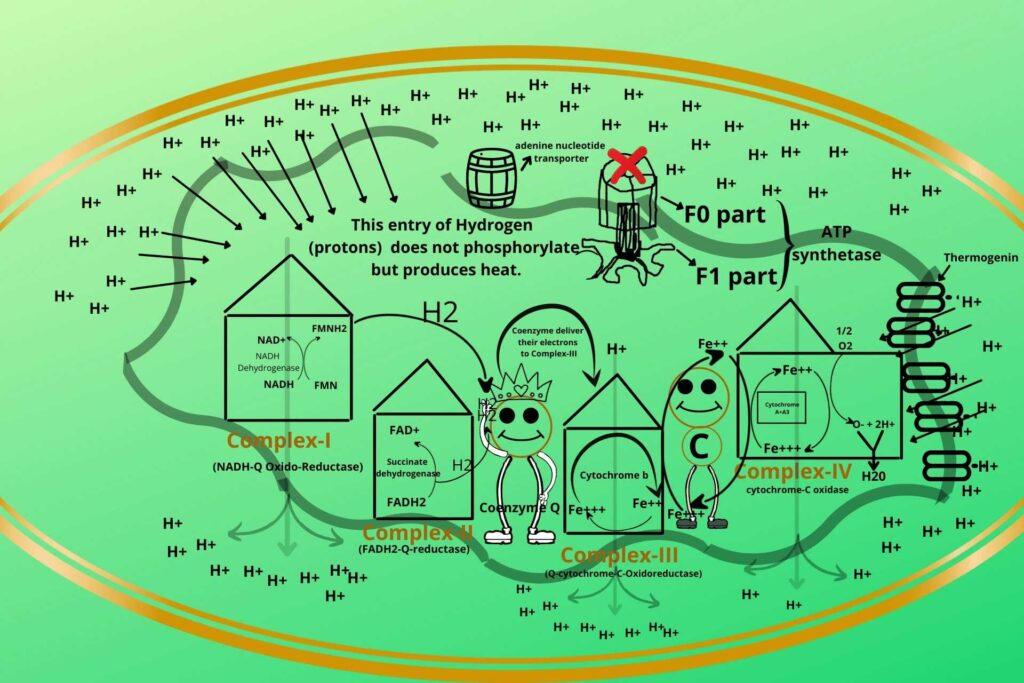
Animals, like the polar bear, when their hibernation period is over and they want to come out then in that time a natural uncoupler called Thermogenin comes (present in their cell) and starts the uncoupling process of Electron Transport Chain (ETC) and oxidative phosphorylation. In this way, a lot of heat is produced and the animal again becomes active.
In human infants, brown fats are present on their back which can produce thermogenin in their mitochondria. The baby is heated and protected from the front by the mother’s breast and chest while the back of the baby has brown fat which produces heating by uncoupling the Electron Transport Chain (ETC) and oxidative phosphorylation and that’s why some cells uncouple the electron transport chain.
That’s super.
Now I am sure you are much more clear about Electron Transport Chain (ETC) and oxidative phosphorylation.
Electron transport chain Summary:
As already discussed Electron transport chain is a chain of reactions in which electrons move through a membrane system to release energy for the conversion of ADP into ATP. First of all, you should know the location of the electron transport chain which is located in the inner mitochondrial membrane. The compounds that enter the electron transport chain are NADH and FADH2 and give their electrons to complex-I and complex-II and again become oxidized. The energy of the electrons passing along the electron transport chain is used to make and transfer H+ from the mitochondrial matrix to the intermembranous space of mitochondria making the intermembranous space negatively charged because a lot of positively charged hydrogen moves out from mitochondria. This creates an attractive force so that H+ enters into mitochondria through ATP SYNTHETASE and gives them the energy to make them rotate, meanwhile, ADP binds to one site of ATP SYNTHETASE and phosphate to another site which after rotation combined together to form ATP.
In last, we concluded that in “electron transport chain and oxidative phosphorylation” ATP is phosphorylated to form ATP while the electron transport chain is an oxidation process, that’s why it is called “electron transport chain and oxidative phosphorylation”.
what you have learned from it write in the comment.
Source:
https://members.drnajeeblectures.com/s/courses/64adb4eae4b061a7c01ce795/take
2 thoughts on “Electron Transport Chain (ETC) and Oxidative Phosphorylation: Relation with Glycolysis and Krebs cycle, Steps with diagrams, and Inhibitors”